Fibers
September 2011 - Note : This is a long overdue revision. Why did this take so long? Because the changes and
insights from so many labs were coming in furiously fast. Some were outright mind numbing. Were they correct? And
some seemed to undo much established knowing. Was there missing stuff that saved the older facts? Some seemed
to be outright contrary to the physical processes as we knew them. Maybe some key steps are missing to make it
right? In fact to make this revision I had to insert my own PRESUMPTION as to certain mechanisms to make a big
piece gel. The latest data seems to support my presumption which was also that of many of those actually doing the work.
One of the huge insights that emerges from the study cellular fibers is that SO MANY, so so many of the syndromes come from messed up fibers.
Didn't we already discuss fibers? Yes. But as this is a pediatric orthopedic site, we need to zoom
out a bit from the atomic to the more grossly cellular. But a brief recap:
Actin filaments are long polymers that wind in twos as a spiral. Actin will be adorned with an
array of other substances so as to make the actin form rope-like clusters or grids that make gels. There are several large molecules which can pull along actin and can drag cellular elements to
destinations along the actin paths.
One such substance, in the cell, is myosin. Myosin has a long tail and a thick head which is composed of several sub units. The head part
can take in ATP which causes it to open its
mouth (and lose its grip on what it is biting), bend at the neck, and bite again. The ATP spring drives this. The presence of ATP and Myosin and its chewy toy - actin - would have myosin nibble its
way to the end of the actin and just sit there able to go no further.
The energy to traverse the actin by using up ATP lacks control. Thus control must be added to this system. As we see it here, the
myosin is a so called Type I, that is loner myosin - single strand type as would be fitting dragging small stuff around inside a cell.
But actin alters its organization and behavior by the company it keeps. Inside the generalized cell it varies by the functional needs from location to location.
But muscle is specialized.
In muscle, the need is that of the colony and not the cell itself. That requirement is external and uniform. Therefore the actin and
myosin of muscle are much more specialized and souped up. We see, above, a piggy back strand (shown in blue) on the actin called tropomyosin. The tropomyosin is fairly straight and stiff. It makes the third strand of the actin/actin/tropomyosin composite rope.
Piggy back on the tropomyosin at intervals is a three part protein whose structure is nearly identical to another cell protein called Calmodulin - a strong attractor of Calcium ion. If there is Ca++ floating about, it will be attracted to the rider on the tropomyosin.
How it almost works:
In a test tube, myosin with ATP will zing to a final end walk position with nowhere else to go. All
you need is actin, myosin and the ATP => boing, it goes. But muscle cells always have actin and myosin and the ATP is nearly always at capacity concentration (a curiosity to be explained later).
The question is how does muscle NOT work? The probability is contraction. The end state is
contraction. Rigor mortis is contraction end state. What makes it not go? Life is controlled improbability.
Well that (blue) strand of tropomyosin does not just coil the actin willy-nilly, it covers most of the
myosin grab points. Myosin can't walk along the actin with those grab points covered. When it is time to contract, the cell dumps a gush of Ca++ into the cell. That calcium ion gets sucked into
the thingy on the tropomyosin (the calmodulin looking thingy), which causes the tropomyosin to roll aside and uncover the actin grab areas. Once those are exposed, myosin does its natural thing
and runs along the actin.
Smooth muscle has actin strung across the cell in X patterns. But in skeletal muscle the structure
is highly parallel and organized as bundles of repetitive parts.
Myosin II, or just plain "myosin" in the context of this discussion, can be grouped like a bouquet
of flowers, as seen in an overly small grouping above (for clarity). But the bundle puts the flowers at both ends.
Bundled myosins are encircled by parallel actin (duel actin + tropomyosin) strands. The actin
attaches mechanically at plates called Z-Plates, named after a famous Spanish American folk hero (or not, it could stand for 'Zah end'). The polarity of actin is such that two groups of actin get
pulled together toward center (M in the middle) as shown by the arrows. There are other fibers whose job is to suspend this stuff.
OK. We have the basic goods. What makes it go? A signal. A signal says "do it" and calcium ion
gets dumped. From where? Around each unit, in a fine mesh-like system of sacs (pink above) called sarcoplasmic reticulum (sarco=muscle, plasm=in the gooey part, reticulum=mesh like - not solid) is a whole bunch of Ca++ just ready to go. On the signal, those membrane spigots open
and the Ca++ floods out into the muscle unit. It contracts.
Skeletal muscle looks like this under a microscope. Each color zone got a designating letter that in
retrospect could have been better thought out. A,B,C or runes, who cares. We now know that this stripe pattern is from the overlap of actin and myosin. The actin ends and attaches at the Z
part. How big the stripes are just reflect the degree of contraction of the muscle as it is observed.
The books all stop here. Story over. Holdit. That can't be all?? OK. Speculation follows. We
know that when whole muscle is stimulated to contract, heat is given off. However, that heat is given off during RELAXATION, it is called heat of relaxation. Whenever heat is given off, generally something important is happening. What? Well for one thing, if those calmodulin-like
tropomyosin thingies so attract calcium ion, how do you get it all back?
You work for it. Pure and simple. It requires a pump and lots of energy to pull that calcium back
into the sarcoplasmic reticulum storage. In a sense, the real energy of muscle contraction is done during relaxation as is the case with a setting cross bow. Work is done to set it up so that it can
spring quickly and powerfully at a moment's notice.
This is very important to us as observers of exercise and big functional activities such as walking.
The further the myosin pulls the actin together, the more work (fuel) will be needed to reset it to starting length.
3 Cases:
1: Stimulus => full unit shortening. The muscle moves something through its full range.
We call this muscle contraction.
2. Stimulus => no shortening at all. The muscle just keeps something where it was.
We call this muscle contraction. uh, wasn't that term already taken...
3. Stimulus => muscle actually elongates. The external object pulls enough to
elongate an activated muscle unit
We call this muscle contraction.
Whoa! That's out and out confusing !!
That's right. The term muscle contraction is essentially USELESS! It has been so muddied by misuse that it no longer means anything.
We will call it muscle activation when a stimulus sets the chemistry in motion. That says nothing
about what actually happens to length. The first case of actual shortening is called concentric muscle activity. The second case, where length is unchanged, is called isometric muscle activity. The last case, the really weird one, the most improbable of the bunch, wherein muscle elongates
in active state, is called eccentric muscle activity.
The biggie? Well, about 99% or better of muscle activity is eccentric in walking - that is in walkers walking.
Didn't expect that, did you? We will visit that in the next section. About that ATP mystery. Why
isn't the ATP consumed? It is - but it is simply replenished pronto by the real energy reserve of muscle called Creatinine Phosphate.
OH
|
C=O
|
H C H
|
H3 C--N H2
|
H N--C=N H
| PO4 (or H) <= This phosphate very quickly recharges the ATP which
is spent down to ADP as the myosin does its AC/DC Angus head flailing walk down the actin. The creatinine Phosphate is what gets depleted. Why this substance? Because it is a fast in-out energized P transport between the muscle filaments area and the goings on in mitochondria where
the high energy gets generated. Those mitochondria are shown in light blue in the image of the actin-myosin functional unit above
Na+ Na+ Na+ Na+ Na+ Na+ Na+
Na+ Na+ Na+ Na+ Na+ Na+ Na+
Na+ Na+ Na+ signal=> Na+ Na+ Na+ Na+
Na+ Na+ =====: Na+ | :=========<: :>========: :=========:= cell membrane K+ | K+ K+ K+ K+ K+ K+ K+ K+ K+
K+ K+ K+ K+ K+ K+ K+ K+ K+ K+
K+ K+ K+ K+ K+ K+ K+ K+
K+
The signal to dump calcium ion is an electrical charge that sweeps along muscle cell membrane.
As the cell membrane sequentially opens pores, potassium (K+) and sodium (Na+) swap places. The potassium concentrated inside the cell, flushes out. Sodium, in higher concentrations outside
, swoops in. The membrane resets by closing those Na/K specific pores and turning on the sodium potassium pumps so as to put things back the way they were.
Most of the creatinine phosphate is ultimately derived , at slow paced activity, from the fat storage mechanism via the cycle described previously. But if pushed to a frenzied pace, the
anaerobic muscle can keep ahead as the citrate cycle mechanism falls off dependent on O2 delivery. Hence higher need for 'carbs' with lactic acid production. Accumulation of lactate also
occurs as removal uses the same limited delivery system as O2 - blood flow..
One interesting outcome of this is that when the overall body mass of muscle is very low, then
what is left works very hard as the load isn't being shouldered by other units. That may result in more lactic acid being produced than would be otherwise expected. We have witnessed the
control of acid base disorder in certain enzyme deficiencies just by increased muscle mass alone ( and subsequent decreased lactic acid acid muscle output). That's not common, but is illustrative.
Can ISOMETRIC muscle action make the whole limb move (in a big way)? answer-> [Yes.]
Collagen & Elastin
The framework that holds us together
.The cloth of which we are made is akin to what we use to dress ourselves. Basically two types
of threads are used. One is tough and makes the cloth that binds the organs and links things together - collagen. The other is stretchy and allows tissues to yield and contour - elastin.
Collagen is made up in many tissues much the same as threads or ropes, with twisted fibers twisted about other twisted fibers.
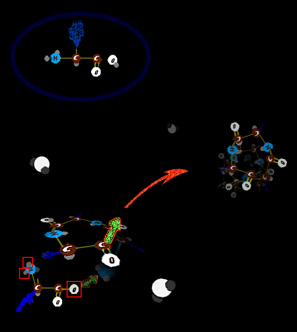
We start with the amino acid core unit. It has a basic C-C-N form (two carbons and a nitrogen). The end nitrogen with hydrogen on the open bonding
areas is the amine (hence amino). The other end ia carbon which has oxygen and hydroxyl terminating its free bonds. That is an acid, -COOH, hence amino 'acid'.
H X HN--C -- C=0 0H
The X
part is different for each amino acid. In the simplest case the X is just hydrogen (that amino acid is called glycine). In more complicated amino acids the X part can be quite involved.
The point is that the N--C -- C portion repeats and becomes the string that links the various pearls:
...N--C -- C--N--C -- C--N--C -- C--N--C -- C--...
and so on. But with a slight curve from one unit to the next, so that a curlycue string evolves (OK, OK, a HELIX. Excusssssssse me!)
The X
part (in the image as a blue smudge) gives the quality to each unit. That x portion may be oily, or something that wets readily (attracts water) or has a high electric charge, or is tangled, or
extends way out and offers a linkage or causes the chain itself to bend a certain way. Nearly a full alphabet of variations make up the alphabet of amino acids. They get strung together the way
letters get strung in a line to make a kind of sense. But note that the string tends to curl up like a slinky. Three amino acids in a row make a near full wind.
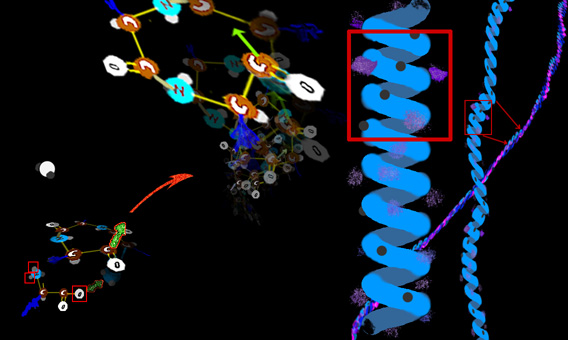 |
The side parts (the X bits) can also interact longitudinally and glue this spiraled chain quite solid.
Even though it begins as a coil, it behaves as a rod. The rod itself isn't exactly straight. It, too has a gentle coil shape. It is called an alpha chain for some excruciating reason.
Every third amino acid in collagen has a nothing X side thingy (just a small hydrogen rather than anything fancy. Called glycine) With all that other stuff sticking out, and with this repeating flat
glycine area (which attracts a certain way), the result is that three of these collagen proteins coil about themselves into a long twisted thread of three protein strings. The multicolor reduced
magnification version is shown above on the right and behind the magnified single strands.
That's collagen. Sort of. Well not just any three proteins twisted. They all have that glycine every
third thing going on. But there are about a dozen variations in how the X parts are configured which alter the way the threads link into a twist (or maybe don't twist at all) or cross link to form sheets.
Think about it. You can have a raccoon tail or a porcupine tail, or cotton candy like structure
depending on the bits that stick out (like bristles from a bristle brush). Hey. That's good. A bristle brush has a central twisted stiff long core and bristles of whatever property is need for the
intended job. Yeah. I like that. Good.
Collagen gets described by naming the three thread subportions. There are three most common alpha chain types. Alpha-1, alpha-2 and guess the next..
yeah [ alpha-3 ] <=highlight to see the tricky answer.
Well, collagen type I is made of two a-1
chains and one a-2. This is the type in bone and tendon and mixed with others in skin and blood vessels.
Collagen type II is made up of three a-1
threads. This collagen is key to cartilage, vertebral disks, and the gooey part of the eye. Those bristles have certain sugar bits attached which really like water.
So, collagen forms strings which are woven through tissue or into ligaments and tendons. But, too
, some versions form sheets. The basement membranes which hold cells in place and which serve as filters are of collagen (types IV and VII).
There are others. Just know that the common thread is - uh - thread.
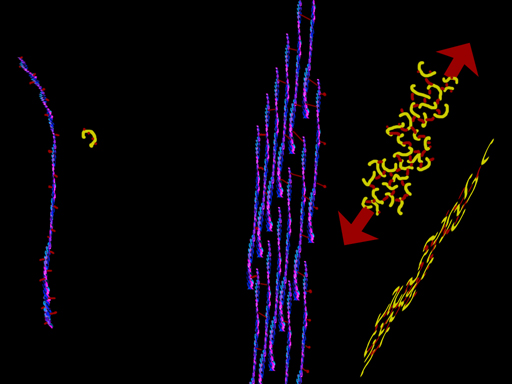
Compare collagen shown in blue with red side thingies, with elastin shown in yellow.
The curly protein shape of elastin proteins and weird
cross links going every which way, allow elastin to spring back after stretching, whereas collagen doesn't give so much.
How are these protein sequences put together? From a recipe! DNA sequences code the sequences for each protein but with handles initially.
STARTGooberGooberThe_Protein_Part_Is_This_PartGooberGooberEND
This gets made then a snip process which recognizes goobers removes the handles:
In the goober handles which get trimmed is code that tells the cell WHERE THIS THING IS TO
GO!!!! It is a mailing address!!! If the protein part is good but the code for the address has an error, guess what? Yeah. The mail isn't delivered.
Think of how many ways this sequence can get muddled. That's about the number of versions of Ehlers-Danlos syndrome there are. If collagen does not work or get to where it has to go, then elastin is used instead.
Ahhh, but there are different kinds of collagens using different alpha chain trios. Sooo, not all collagen locations are affected, right?
Right. Sometimes vessels are way bad and other times not. Sometimes the skin falls apart and other times not too bad.
But what happens if the handles of the collagen don't get snipped off so that the cell can expell
the final product? Well if that defect is happening to type I collagen, we have osteogenesis imperfecta
- brittle bones disorder. Remember bone is not calcium. Calcium (as a crystal called hydroxy appetite) merely hardens the cloth we call bone. Bones depleted of calcium by acid can
be tied in knots - but they are still bones. However, without the fiber basis, the core collagen framework, it becomes chalk - brittle.
If the collagen can't nestle together because a glycine (having no stickyout thingy) gets substutited
by an amino acid with aside bit sticking out, then the coil gets screwed up. How bad the inability to coil the triple protein thread or how deficient the delivery determines how severe the
manifestation. That's why we see all shades of this from a wee bit easier to break when skiing to in utero lethal.
OK, now it gets tricky.
We know some new stuff. OBVIOUSLY - Marfan syndrome has got to be an elastin disorder as
all the bits of the syndrome are from missing properties of elastin.. Right? Well, it turns out that in Marfan's patients, there have been identified (as of this moment) over 650 different genetic
alterations which can mess up a fiber called FIBRIN ????? [Hey! What's fibrin? You never mentioned FIBRIN!]
OK, we have to get phylogeny in here. Elastin, on the tree of animal species, is a newcomer. Fibrillin is the generic gooey fiber that holds non-mega-strong stuff in a general blob organization (strong stuff being ligaments, tendons, bone etc). But climbing the animal kingdom tree, starting
with things that pump - vascular systems with a heart - where pulses not only happen but need to transfer pressure waves - neither the Herculean collagen nor the jelly fish fibrin will do the job.
Elastin appears. Elastin isn't just curly, go look at that image again. It is cross linked to make maximum use of the curvature and its springy nature.
Elastin is rich in cysteine (which has sulphur in its side group) which are beautifully suited to
sulfur mediated cross attachment (cysteine-cysteine) and also hydroxy-lysine amino acids which are able to use vitamin-C mediated hydroxyl cross links (hydroxylysine-hydroxylysine). This is
essential to arteries such as the aorta (Marfan defect is aneurysm or ballooning out) as well as eye lens support and control (Marfan defect is lens dislocation etc). There are a variety of limb
proportionality issues as well. So fibrillin? The defect is in fibrillin?
It seems that way - but as fibrillin, a fiber-like material found in the stuff between cells has been
around much longer and seems not so much affected by subtle amino acid variation in species that don't have elastin, the thinking is that elastin uses the fibrillin (which does tend to glom onto
elastin in certain experiments) to set the actual elastin cross link points. In other words, elastin uses fibrillin as a template for its own cross linking. An alternative is that the two TOGETHER
create the required structural entity. Defects of fibrillin do not always seem to bother unique fibrillin jobs so much as they do the exact patterns elastin requires to cross link properly. Thus,
other sources of metabolic error (homocysteinurea) would also mess up elastin cross linking and produce a syndrome that looks exactly like Marfan syndrome - but minus the fibrillin defect.
And yes. Of course there is more than one type of fibrillin. Fibrillin is more a group name of
fibers so designated by their length as they filter out in the lab. This group of stringy molecules are not just long but they also contain portions which are called 'growth factor like' or
transformation zones wherein they have some affect on cell life and transformation and thus under the eye of the scientists looking for cancer causes and cures. This story is rapidly
expanding by the day. Almost any 'fact' in this last section is up for grabs as it needs to be sorted out if the properties of these things held in isolation are like or unlike their behavior when paired
with other structures (like a steering wheel with no steering column).
So what gene does what disease?
If you want to curl up with a cozy read on a cold rainy evening, may I suggest:
Victor A. McMcKusick 's Mendelelian Inheritance in Man (12th ed). Volume 3 ends on page 3612 with section: 508500. I won't tell you the ending (has to do with Wolfram's syndrome,
mitochondrial form). But you'll never guess what 4p16 predisposes to.
|